Experimental proof of lamellar magnetism using polarised neutrons
Back in 2002, I was part of a team that proposed a radically new mechanism for generating magnetic remanence in the ilmenite-hematite system (Robinson et al. 2002). The so-called “lamellar magnetism” hypothesis was put forward to explain the unusually strong and stable magnetisation of slowly-cooled rocks containing intergrowths of ilmenite and hematite. The most puzzling feature of the rocks was that they were much more magnetic than expected. Hematite is an antiferromagnet, which means its magnetic structure consists of two sublattices of opposing magnetic spins. In a perfect antiferromagnet, the opposing spins cancel each other out exactly, leading to zero net magnetic moment. In hematite, however, a slight misalignment (or canting) of its spins leads to a very weak net magnetic moment that points in a direction perpendicular to the sublattice spins (red arrow in figure below). Ilmenite is a paramagnet, which means its magnetic spins are thermally disordered and cannot contribute at all to the observed magnetisation. This mixture of weakly magnetic and non-magnetic minerals left us with the paradox of a rock who’s magnetisation was far greater than the sum of its parts!
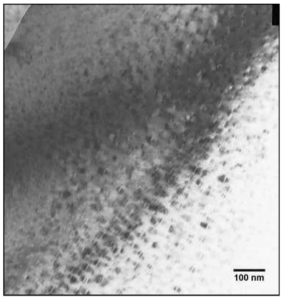
Bright-field transmission electron micrograph of a natural intergrowth of nanometer-scale hematite precipitates (bright lines surrounded by dark shadows) within a matrix of ilmenite. A different crystal from the same sample was used for the neutron diffraction work.
The key to unlocking the mystery of the excess magnetisation was the realisation that the intergrowths of ilmenite and hematite occurred on extremely fine length scales. Transmission electron microscopy revealed that lamellae of ilmenite in hematite (and vice versa) continued right down to the unit cell scale (~ 1 nm). A key principle in nanotechnology is that the properties of materials can change fundamentally at the nanometre scale, partly due to the increasing dominance of surfaces and interfaces. Our hypothesis was that the excess magnetic moment originated from a local imbalance of magnetic moments at the interfaces between nanoscale ilmenite and hematite lamellae (blue arrow in figure below). This excess lamellar moment can be thought of as a form of “defect” moment that is generated every time a nanoscale precipitate is placed inside its host. Crucially, this defect moment was predicted to be oriented parallel to the sublattice magnetisation of hematite, in contrast to the perpendicular orientation of a canted moment.
This prediction lead to a simple experimental test that could prove or disprove the lamellar magnetism hypothesis: take a single crystal sample containing a fine-scale intergrowth of ilmenite and hematite, apply a saturating magnetic field (i.e. one that is large enough to cause the net moment throughout the entire sample to align with the field) and measure the corresponding orientation of the sublattice spins with respect to the field. There are three possible outcomes of the experiment: 1) if the sublattices adopt an orientation parallel to the field then the net moment is generated by lamellar magnetism alone, 2) if the sublattices adopt an orientation perpendicular to the field than the net moment is generated by canting alone, 3) if the sublattices adopt an intermediate orientation, then both lamellar magnetism and canting contribute to the net moment.
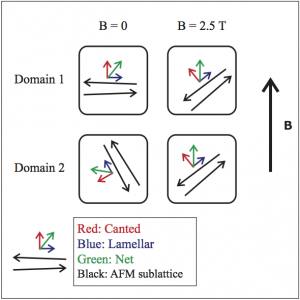
By measuring the angle between the sublattice spins (black) and the net magnetisation (green) in a saturating field, the presence of both a canted moment (red) and lamellar moment (blue) can be detected.
Our paper published this week in Physical Review B used polarised neutron scattering to perform this test at last. Neutrons are a sensitive probe of magnetism since they themselves possess a spin, causing them to be scattered by the magnetic moments of the atoms in a sample. If the spin of an incoming beam of neutrons is oriented perpendicular to the atomic moments, then the scattered beam of neutrons will have its spin direction reversed (or ‘flipped’). If the spin of the incoming beam is oriented parallel to the atomic moments in the sample, then the scattered neutrons will keep their original spin direction. By measuring the relative intensity of the ‘spin flip’ versus ‘non spin flip’ signals, the orientation of the sublattice spins can be deduced. The results presented by Brok et al. (2014) demonstrate that the sublattices adopt an average angle of ~ 60° to a saturating field (i.e. option 3 of the possible outcomes described above). This simple (yet extremely hard-won) result is the most direct demonstration yet that lamellar magnetism contributes significantly to the magnetic properties of ilmenite-hematite intergrowths, and a fantastic example of nanopaleomagnetism in action.
Richard Harrsion
Robinson, P., Harrison, R.J., McEnroe, S.A., and Hargraves, R.B. (2002) Lamellar magnetism in the haematite-ilmenite series as an explanation for strong remanent magnetization. Nature, 418, 517–520.
Brok E, Sales M, Lefmann K, Theil Kuhn L, Schmidt WF, Roessli B, Robinson P, McEnroe SA and Harrison RJ (2014) Experimental evidence for lamellar magnetism in hemo-ilmenite by polarized neutron scattering. Physical Review B, 89, 054430.
Leave a Reply