Meteorites capture the magnetic field of the solar nebula
Nanopaleomagnetism of dusty olivine provides experimental evidence that strong magnetic fields shaped the early solar system
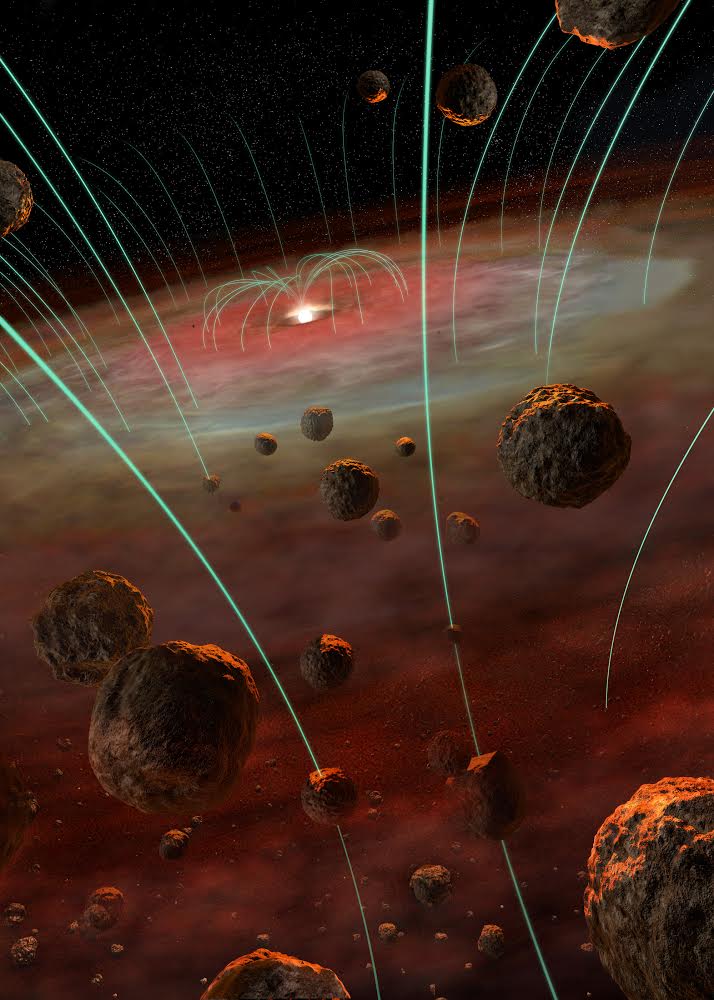
Artist’s impression of chondrules being exposed to the nebular magnetic field. Image Credit: Hernán Cañellas. Copyright MIT Paleomagnetism Laboratory (reproduced with permission).
The early solar system was a violent and dynamic place. Shortly following the birth of the Sun, the solar nebula transformed from a cloud of dust and gas into a rapidly evolving accretionary disk, in which clumps of dust particles were being flash heated to temperatures up to 2000 K. Heating caused these dust balls to melt, forming spherical droplets that cooled and recrystallised into chondrules – the mm-sized rounded particles that are the defining feature of chondritic meteorites (the building blocks of the Earth). The precise cause of the heating events that lead to the formation of chondrules is one of the most important outstanding questions in cosmochemistry. Both the mechanism and location of chondrule formation can be constrained by measuring the intensity of the magnetic field that chondrules were exposed to as they crystallised and cooled. Some proposed mechanisms involve formation close to the early Sun, implying that chondrules would have been exposed to strong magnetic fields as they cooled. Other mechanisms (such as nebular shock waves) would be consistent with weaker magnetic fields. The nebular magnetic field plays a crucial role in the inward transport of material and outward transport of angular momentum in the early solar system, yet until now there have been no reliable experimental observations of nebular field intensity. A new study published today in Science describes the first experimental observations of the nebular magnetic field extracted from measurements chondrules from the Semarkona meteorite – the culmination of several years of collaboration between teams of paleomagnetists at M.I.T. (lead by Ben Weiss) and mineralogists at Cambridge (lead by Richard Harrison). The results not only provide dramatic new insights into the processes that shaped the early solar system, but provide a perfect illustration of how nanopaleomagnetic methods can be used to help tackle one of the most challenging problems in paleomagnetism – the quantification of pre-accretionary remanence.
NPMs involvement in the project began back in 2011 when Harrison, PhD student Sophie Lappe and post doc Nathan Church started work on the fundamental question of whether chondrules were capable of recording and retaining reliable pre-accretionary remanence. Only minerals with the highest levels of magnetic, thermal and chemical stability are likely to be able to prevent their primary remanence from becoming corrupted or erased during the meteorite’s long journey to Earth – a journey that may have included thermal and chemical processing on the meteorite’s parent body, impact shocks due to ejection from the parent body, transport through Earth’s atmosphere, weathering on Earth’s surface, and potential exposure to magnetic fields during collection and subsequent sample storage. Inspired by an experimental paper by Minoru Uehara, the Cambridge team began to investigate the properties of ‘dusty olivine’: grains of olivine with a ‘dusty’ appearance caused by the presence of nanoscale particles of metallic iron. These particles formed via subsolidus reduction of the ferrous iron component of the olivine host during flash heating in the solar nebula. By examining the magnetic state of the iron particles using electron holography, they were able to conclude that dusty olivine did indeed have what it takes to hold onto a magnetic signal for 4.5 billion years (Lappe et al. 2011). The high fidelity of dusty olivine as a paleomagnetic recorder is partly due to the small size of the iron particles, which leads to the formation of magnetically and thermally stable single domain and single vortex states, and partly due to the protective effects of the surrounding silicate, which prevents alteration or oxidation of the delicate magnetic remanence carriers. This work was followed by a detailed investigation of the rock magnetic properties of dusty olivine, resulting in the methods and calibrations needed to convert the magnetic memory of individual chondrules into reliable estimates of the nebular magnetic field (Lappe et al. 2013).
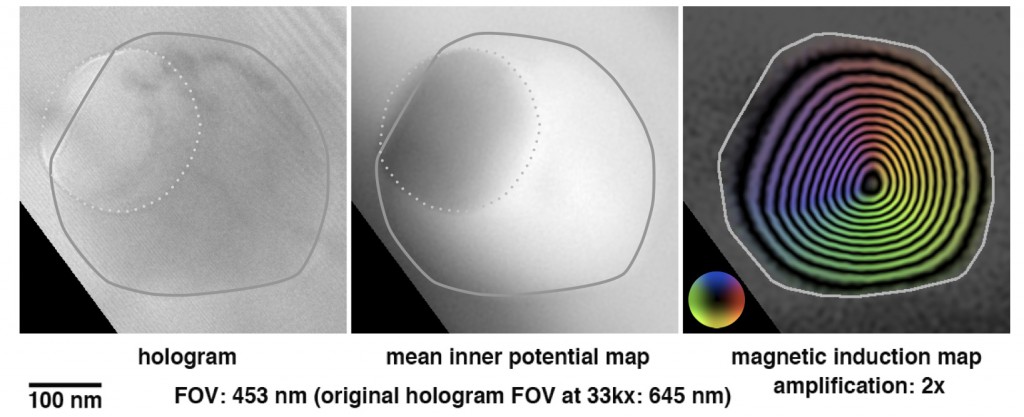
Electron holography showing single vortex state in a dusty olivine particle from the Semarkona meteorite used in the study.
The scene was set to attempt measurements of the remanence of dusty-olivine bearing chondrules in meteorite samples. Two key technical challenges had to be overcome, however. Firstly, regions of dusty olivine are incredibly small, typically only occurring in patches ~ 100 µm in size, located inside individual chondrules. Secondly, the magnetic signals from dusty olivine are incredibly weak. The only instrument capable of measuring such small signals is a scanning SQUID microscope – a highly sensitive magnetometer that can measure the magnetic field emanating from a polished sample with incredible precision and ~100 µm spatial resolution. Unfortunately, even this high resolution was insufficient to enable the weak signals from the dusty olivine to be separated from the stronger signals coming from large magnetic particles around the rim of the chondrule. All attempts to mathematically separate the signals failed, and we needed to think again.
At this point Roger Fu, PhD student at M.I.T., hit upon the idea that if the signals could not be mathematically separated, the only other option would be to physically separate them. Using a precision dentist’s drill mounted on an optical microscope, Fu painstakingly scraped away the rims of each chondrule, leaving behind isolated and pristine regions of dusty olivine. He was now able to use the SQUID microscope to measure the magnitude and direction of the magnetic remanence of each region of dusty olivine, and subject the meteorite to a series of stringent paleomagnetic tests in the search for pre-accretionary remanence. The crucial observation was that each chondrule measured had a different remanence direction (a so-called “positive conglomerate test”). This is a necessary result if the chondrules were magnetised prior to their accretion onto the parent body (if the meteorite had been magnetised after accretion, all chondrules would have the same remanence direction). Passing the conglomerate test is crucial evidence in support of pre-accretionary remanence, but it is not definitive. False positives have been reported in the past, especially when the non-uniform magnetisation of the chondrule rims is not taken into account. Fu, however, went one step further. Using the dentist’s drill he was able to split several chondrules in two and measure each half separately. Each half gave the same magnetisation direction, demonstrating that the interior of each chondrule is uniformly magnetised, ruling out the possibility of a false positive. Combining the remanence values with the calibrations worked out by Lappe, the first measurement of the nebular magnetic field of 51 ± 24 µT was obtained.
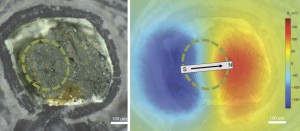
Dusty olivine grain isolated from a chondrule using a nonmagnetic micro drill. (b) Dipolar magnetic stray field measured using scanning SQUID microscopy, enabling the magnitude and direction of magnetisation of the dusty olivine grain to be measured.
Based on this result it is likely that chondrules formed either as molten droplets resulting from the collisions of 10- to 1,000-kilometer rocky bodies, or through the spontaneous compression of surrounding gas, which melted dust particles together. It is unlikely that chondrules formed via electric currents, or X-wind — flash-heating events that occur close to the sun. According to theoretical models, such events can only take place within magnetic fields stronger than 100 µT.
For further information on the implications of the results, see the summaries provided by other co-authors.
M.I.T. Press Release
Pulling together the early solar system: New study finds that a strong magnetic field whipped the early solar system into shape.
A.S.U. Press Release
Magnetic fields frozen into meteorite grains tell a shocking tale of solar system birth
A link to the paper can be found on our publications page.
Leave a Reply