World’s first X-ray Holography Measurement of an Iron Meteorite Performed at BESSY II
Magnetic imaging is one of the central tasks in nanopaleomagnetic research. A number of Transmission Electron Microscopy (TEM) methods for magnetic imaging with nanoscale spatial resolution are available (e.g. Electron Holography, Lorentz Microscopy, Differential Phase Contrast imaging). However, they all suffer from one key drawback: electrons are strongly deflected by applied magnetic fields, so measurements normally have to be performed under near field-free conditions – great if you want to image remanence states, but not so great if you are interested in hysteresis properties…
The problem of magnetic imaging in strong fields can be solved by using X-rays instead of electrons to probe the magnetic state of the sample. X-rays are unaffected by magnetic fields, but this freedom comes at a price: unlike electrons, X-rays cannot be focussed using electromagnetic lenses, making imaging with X-rays a particular challenge.
X-ray holography is a high-resolution imaging technique that circumvents the need for lenses entirely. Instead it uses coherent diffraction from the sample and a known reference object to form a holographic interference pattern. Using Fourier transforms, an image of the sample (convoluted with the reference object) is extracted from the hologram. This approach yields spatial resolution down to 30 nm, and the ability to image in applied magnetic fields up to 2 Tesla.

Principle of X-ray holography, as pioneered by Eisebitt et al. (2004) Lensless imaging of magnetic nanostructures by X-ray spectro-holography. Nature 432, 885-888.
To perform an X-ray holography experiment, the sample (normally a thin film, but in our case a < 100 nm thick TEM lamella) is placed on a silicon nitride membrane. The reverse side of the membrane is coated in 600 nm of gold, which is opaque to soft X-rays. A circular window, 5 µm in diameter, is milled into the gold mask, exposing the region of the sample to be imaged. A small reference hole (~30-80 nm in diameter) is drilled adjacent to the sample window. The sample and reference are illuminated with a coherent beam of circularly polarised soft X-rays, tuned to the Fe L3 absorption edge, and the resulting holographic interference pattern is recorded on a high-resolution CCD camera. The inverse Fourier transform of the interference pattern is calculated and the image of the sample extracted from one of the resulting side bands. The magnetic signal is separated from non-magnetic contributions by subtracting the left- and right-circularly polarised images, yielding dark or light contrast which is proportional to the out-of-plane component of magnetisation.
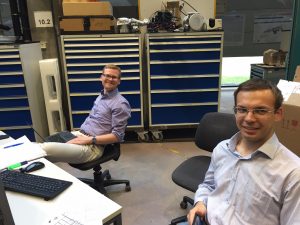
Bastian Fau and Roberts Blukis: searching for high resolution hysteresis measurements using X-ray holography.
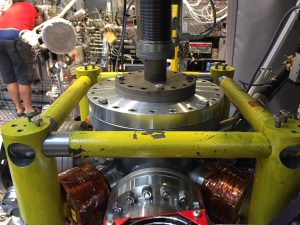
Close-up of the sample chamber. A series of 4 coils (two visible in the lower part of the image) allow magnetic fields up to 2 T to be applied in directions parallel, perpendicular and at 45° to the sample plane.
Collaborating with X-ray holography experts Bastian Fau, Christian Günther and Stefan Eisebitt, we recently applied this method for the first time to image the nanomagnetic state of the cloudy zone in an iron meteorite at the synchrotron BESSY II in Berlin. The cloudy zone is a nanoscale intergrowth containing islands of tetrataenite in an Fe-rich matrix. The high coercivity and dense packing of tetrataenite islands makes it a natural target for nanopaleomagnetic studies, but we know little about how the cloudy zone responds to strong magnetic fields, and in particular how magnetic interactions between adjacent islands might influence their collective behaviour. Using X-ray holography we were able to image the switching behaviour of the cloudy zone as the magnetic field was swept from + 1 T to -1 T and back again (click on the image below to see the movie). The movie shows the sequence of magnetic states obtained through a full hysteresis cycle. Switching of individual tetrataenite islands and groups of islands can be seen as a sudden change from black to white contrast. Sweeping of magnetic domain walls across the tetrataenite rim are also clearly resolved, as well as evidence for the interaction of magnetic domain walls and crystallographic twin walls in the rim.
We are only just beginning the process of analysing these measurements. Eventually we hope to extract hysteresis loops from individual tetrataenite islands, and examine them for evidence of inter particle interactions. Comparison of the observations with micromagnetic simulations will help us understand the underlying physics of these unique nanostructures, with the ultimate aim of deciphering the true nature of the magnetic signals they acquire during formation on the meteorite parent body. In the mean time, enjoy this sneak peak of what can be achieved using X-ray holography – watch this space!
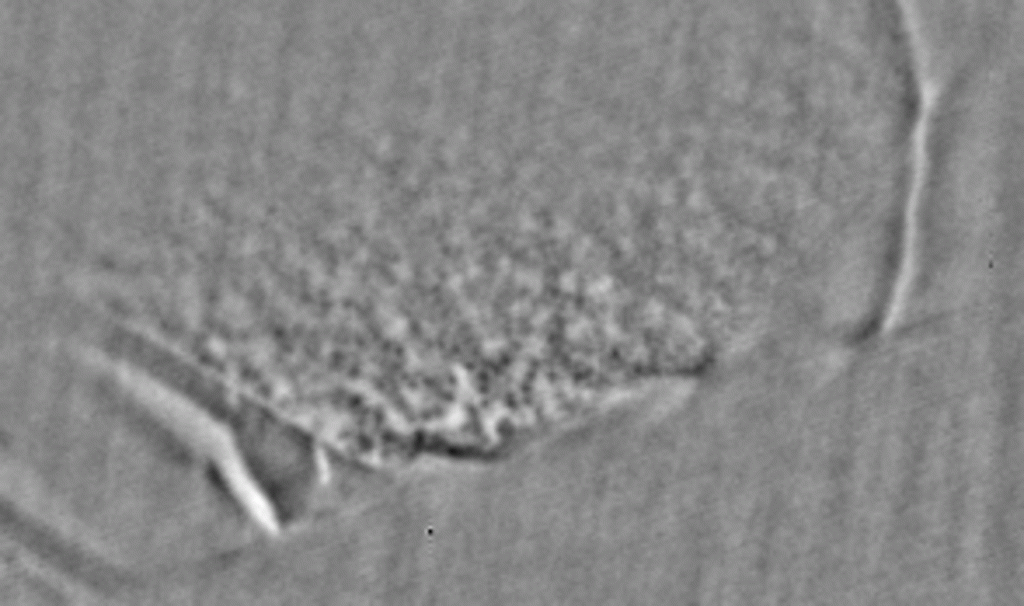
X-ray holographic image of the cloudy zone. The horizontal field of view is 5 µm. Dark and light regions correspond to magnetisation pointing either into or out of the plane of the sample. The region containing large magnetic domains on the left corresponds to the tetrataenite rim. The fine-scale features correspond to the island/matrix structure of the cloudy zone, with the size of the islands decreasing from left to right across the image. CLICK ON THE IMAGE TO SEE THE MOVIE!
Leave a Reply